Home » News & Events » Reducing Wind Energy Cost through Increased Turbine Size
Reducing Wind Energy Cost through Increased Turbine Size
The growing size of wind turbines has helped lower the cost of wind energy to the point that it is economically competitive with fossil-fuel alternatives in some locations. But can turbines continue to scale in the future, or are they running into physical or logistical constraints? Recent research published in the journal Nature Energy suggests that land-based wind turbines and, especially, offshore turbines have room to grow, offering the promise that this already-mature energy technology will see still lower costs in the future.
The new study summarizes a global survey of 163 of the world’s foremost wind energy experts to gain insight into the possible magnitude and sources of future wind energy cost reductions. It represents the largest-ever “expert elicitation” survey on an energy technology, and was led by Berkeley Lab, NREL, University of Massachusetts, and participants in the IEA Wind Technology Collaboration Programme Task 26.
According to the wind experts, larger turbines are on the horizon, enabling further reduction in the cost of wind energy on land and offshore. Turbine design will continue to vary by market and project site, but the experts forecast continued evolutionary growth in average turbine size on land, and more revolutionary growth offshore. Figure 1 depicts the historical growth in land-based turbine size in the United States, while also presenting survey-based average forecasted turbine size in North American in 2030. Figure 2, meanwhile, shows historical global offshore turbine size trends and survey-based average forecasted size globally, also in 2030.
Turbine Design Optimization in an Historical Context
The story of wind power is a story of machine size. From the beginnings of the U.S. industry in California to the present, the typical generation capacity of wind turbines deployed in the United States has increased 20 fold, from the 100 kW turbines typical in the 1980s to the 2 MW machines common today. Modern turbines feature 80 meter towers, and 100 meter rotor diameters: big enough to sweep an area 50 percent larger than an American football field.
With that scale has come both decreased up-front costs (partly through economies of scale and reduced balance-of-plant costs on a per megawatt basis) and increased turbine performance (through taller towers and longer blades). In combination, these trends have reduced the levelized cost of wind energy (LCOE).
Of course, turbine size varies by market, by wind resource and site topography, and by whether turbines are deployed on land, or at sea. There is no single “optimal” turbine across all sites and, as technology has developed, turbine designs have also shifted.
As documented in the DOE’s 2015 Wind Technologies Market Report, the most notable recent trend in the United States on land has been towards longer blades and the resultant increase in swept rotor area (also see Figure 1). Yet average turbine generator capacity has stayed roughly the same since 2011. In other words, companies are attaching bigger blades to the same generator size, thus increasing energy capture. And because the additional up-front capital cost of doing so is modest, the overall trend has been towards a lower overall levelized cost of wind energy. The ratio of generator size to rotor swept area is known as “specific power,” and is an important and underappreciated development in wind technology.
Though the new generation of low specific power machines was originally designed for lower wind speed sites, they are now being deployed in some of the windiest parts of the country. This is enabled by the low turbulence winds often seen in the U.S. interior and by improved turbine materials and control systems. The result: the average capacity factor of recently built projects exceeds 40%, a level unheard of even a few years ago (Figure 3).
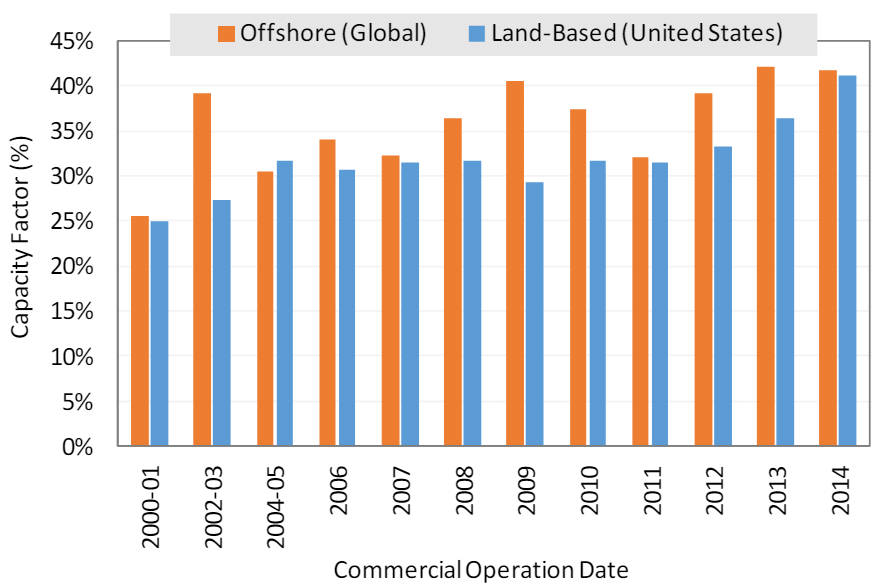
Figure 3
Specific power has declined in other markets, but the U.S. is unique in the speed and degree of decline. In 2015, average turbines installed in the U.S. had a specific power of 250 W/m2, compared to 330 W/m2 in Germany and 350 W/m2 in Denmark. In contrast, many European markets feature larger average generator ratings (2 MW in the U.S. in 2015, compared to 2.7 MW in Germany and 3.1 MW in Denmark). And some markets, most notably Germany, have seen far greater growth in hub heights (82 m in the U.S. in 2015, 123 m in Germany).
These trends are, in part, related to the limited number of developable high-quality wind resource sites that exist in much of densely-populated Europe, constraints that support the deployment of turbines that maximize energy production from every single turbine pad. The tall and high capacity turbines that meet these needs do not yet generally feature the outsized rotors (and low specific power) that have become common on smaller turbine platforms. As design and logistical challenges to longer blades are addressed, this may change.
Offshore, the wind sector is less mature. Nonetheless, the 1.6 MW global average size of turbines installed in 2000 has given way to an average size of more than 4 MW in 2015 (Figure 2). Hub heights and rotor diameters have similarly increased, with a modest trend towards lower specific power and with a resultant increase in capacity factors (Figure 3, also see here).
Offshore turbines will continue to grow, with most projects in planning today expecting to use turbines in the 6-8 MW range. In part this is due to the easier logistics of transporting massive components by sea. But, in addition, the non-turbine costs of building offshore wind plants (and especially the cost of the foundation and installation) are enormous. Minimizing those costs on a per-MW basis through growth in turbine size is a decided advantage.
Reducing Costs, Enhancing Value, and Increasing Developable Areas with Turbine Design
The increasing size of wind turbines is a core contributor to lower LCOE, according to the experts in the survey. The cost of land-based wind is expected to fall by, on average, 24% by 2030 and 35% by 2050 under the median (best guess) scenario. Fixed-bottom offshore cost declines are even greater, at 30% by 2030 and 41% by 2015. And costs could be lower for both applications: experts predict a 10% chance that costs will decline by more than 40% by 2030 and more than 50% by 2050.
Though many factors can drive energy cost reductions, turbine size is key. Experts assessed 28 different possible means of reducing LCOE, with the top 5 listed in Table 1. Land-based wind benefits most from increased rotor size (and reduced specific power), rotor design advancements, and increased tower heights. For fixed-bottom offshore wind, the single largest driver is increased turbine generator capacity ratings and rotor diameters, with specific power maintaining current levels.
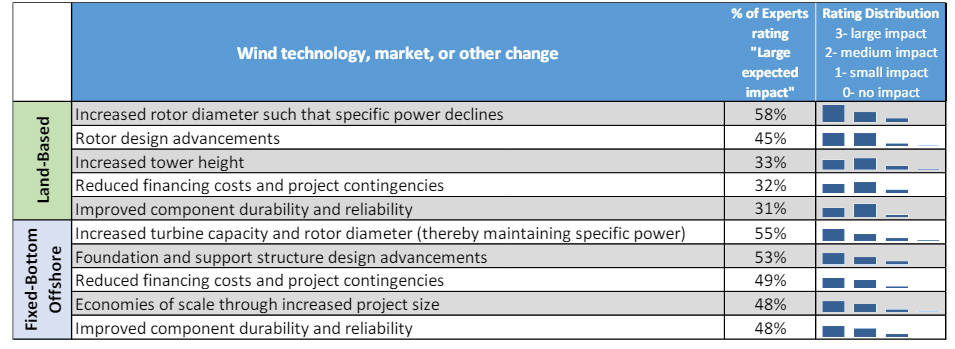
Table 1
The survey also asked how reductions in up-front capital costs (CapEx) and operating expenses (OpEx), increases in capacity factors and turbine design life, and improvements in the cost of financing would affect energy costs.
For land-based wind, experts anticipate that LCOE reductions will be primarily influenced by improvements in capacity factors and CapEx. The taller towers and larger rotors expected to be deployed by 2030 are consistent with the relative importance placed on capacity factor improvements. Achieving lower capital costs while simultaneously increasing turbine size, meanwhile, will require continued design, engineering, and manufacturing advancements.
For fixed-bottom offshore wind, reduced upfront costs are seen as the single most-important driver for LCOE improvements to 2030, with capacity factor increases of somewhat lesser significance. Larger average turbine generator capacities will reduce the high (per-MW) cost of substructures, foundations, and installation experienced offshore. As a report by NREL puts it, “Fewer larger sized turbines minimize the balance-of-system requirements (i.e., less substructures and other infrastructure required to achieve the same project size).” A recent report by BVG Associates, meanwhile, highlights the positive impact of turbine generator size, hub height, and rotor length on not only CapEx, but also OpEx and capacity factor; that report cites a possible 18% reduction in LCOE between 2014 and 2030 based on turbine size alone.
Lower energy costs are not the only benefit from bigger turbines. Recent research shows that machines with low specific power (and taller towers) can boost the value of wind energy in electricity markets, since they have steadier output. Work by and for the U.S. Department of Energy, meanwhile, shows how larger turbines and turbine design advancements might unlock new areas for wind development, both on land and offshore, that would otherwise be deemed unattractive.
The new study summarizes a global survey of 163 of the world’s foremost wind energy experts to gain insight into the possible magnitude and sources of future wind energy cost reductions. It represents the largest-ever “expert elicitation” survey on an energy technology, and was led by Berkeley Lab, NREL, University of Massachusetts, and participants in the IEA Wind Technology Collaboration Programme Task 26.
According to the wind experts, larger turbines are on the horizon, enabling further reduction in the cost of wind energy on land and offshore. Turbine design will continue to vary by market and project site, but the experts forecast continued evolutionary growth in average turbine size on land, and more revolutionary growth offshore. Figure 1 depicts the historical growth in land-based turbine size in the United States, while also presenting survey-based average forecasted turbine size in North American in 2030. Figure 2, meanwhile, shows historical global offshore turbine size trends and survey-based average forecasted size globally, also in 2030.
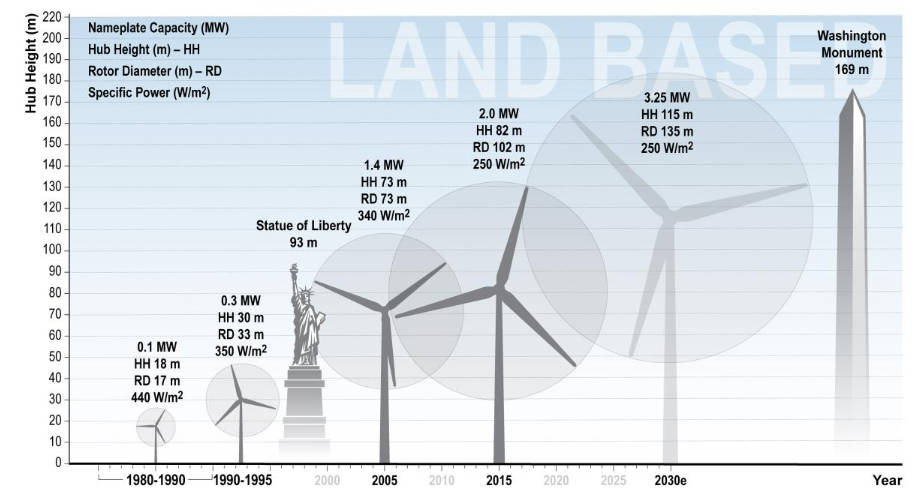
Figure 1
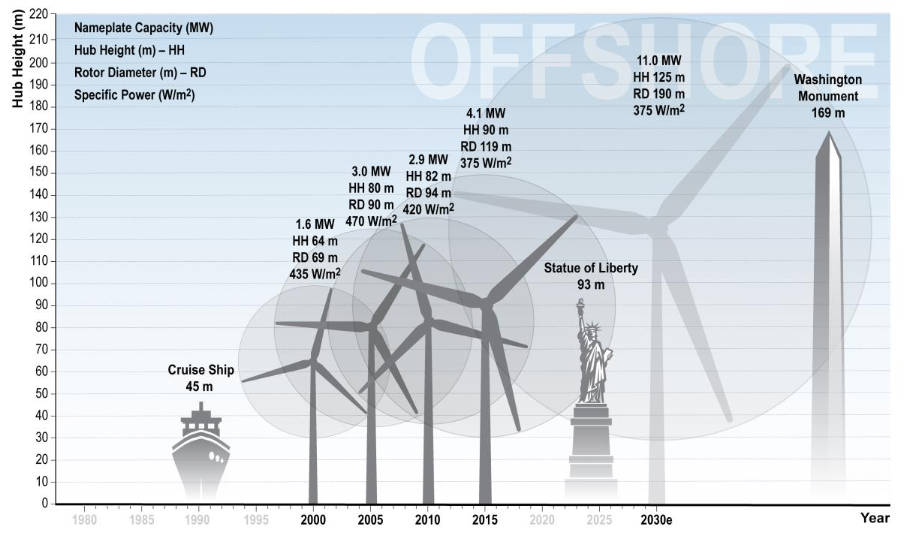
Figure 2
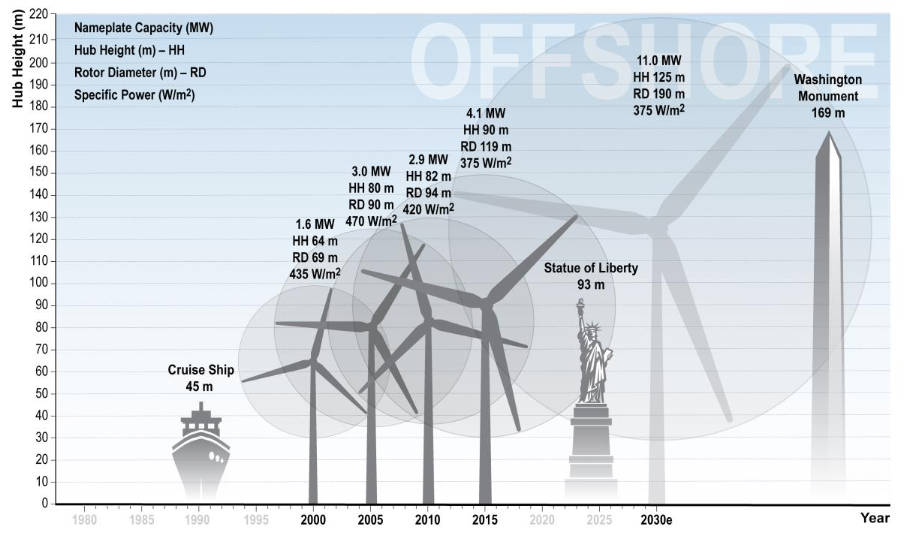
Figure 2
Turbine Design Optimization in an Historical Context
The story of wind power is a story of machine size. From the beginnings of the U.S. industry in California to the present, the typical generation capacity of wind turbines deployed in the United States has increased 20 fold, from the 100 kW turbines typical in the 1980s to the 2 MW machines common today. Modern turbines feature 80 meter towers, and 100 meter rotor diameters: big enough to sweep an area 50 percent larger than an American football field.
With that scale has come both decreased up-front costs (partly through economies of scale and reduced balance-of-plant costs on a per megawatt basis) and increased turbine performance (through taller towers and longer blades). In combination, these trends have reduced the levelized cost of wind energy (LCOE).
Of course, turbine size varies by market, by wind resource and site topography, and by whether turbines are deployed on land, or at sea. There is no single “optimal” turbine across all sites and, as technology has developed, turbine designs have also shifted.
As documented in the DOE’s 2015 Wind Technologies Market Report, the most notable recent trend in the United States on land has been towards longer blades and the resultant increase in swept rotor area (also see Figure 1). Yet average turbine generator capacity has stayed roughly the same since 2011. In other words, companies are attaching bigger blades to the same generator size, thus increasing energy capture. And because the additional up-front capital cost of doing so is modest, the overall trend has been towards a lower overall levelized cost of wind energy. The ratio of generator size to rotor swept area is known as “specific power,” and is an important and underappreciated development in wind technology.
Though the new generation of low specific power machines was originally designed for lower wind speed sites, they are now being deployed in some of the windiest parts of the country. This is enabled by the low turbulence winds often seen in the U.S. interior and by improved turbine materials and control systems. The result: the average capacity factor of recently built projects exceeds 40%, a level unheard of even a few years ago (Figure 3).
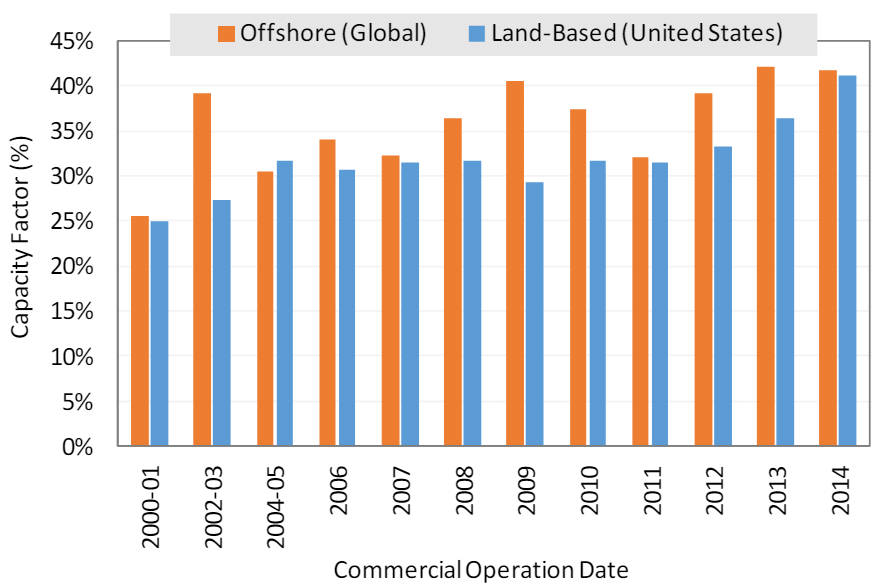
Figure 3
Specific power has declined in other markets, but the U.S. is unique in the speed and degree of decline. In 2015, average turbines installed in the U.S. had a specific power of 250 W/m2, compared to 330 W/m2 in Germany and 350 W/m2 in Denmark. In contrast, many European markets feature larger average generator ratings (2 MW in the U.S. in 2015, compared to 2.7 MW in Germany and 3.1 MW in Denmark). And some markets, most notably Germany, have seen far greater growth in hub heights (82 m in the U.S. in 2015, 123 m in Germany).
These trends are, in part, related to the limited number of developable high-quality wind resource sites that exist in much of densely-populated Europe, constraints that support the deployment of turbines that maximize energy production from every single turbine pad. The tall and high capacity turbines that meet these needs do not yet generally feature the outsized rotors (and low specific power) that have become common on smaller turbine platforms. As design and logistical challenges to longer blades are addressed, this may change.
Offshore, the wind sector is less mature. Nonetheless, the 1.6 MW global average size of turbines installed in 2000 has given way to an average size of more than 4 MW in 2015 (Figure 2). Hub heights and rotor diameters have similarly increased, with a modest trend towards lower specific power and with a resultant increase in capacity factors (Figure 3, also see here).
Offshore turbines will continue to grow, with most projects in planning today expecting to use turbines in the 6-8 MW range. In part this is due to the easier logistics of transporting massive components by sea. But, in addition, the non-turbine costs of building offshore wind plants (and especially the cost of the foundation and installation) are enormous. Minimizing those costs on a per-MW basis through growth in turbine size is a decided advantage.
Reducing Costs, Enhancing Value, and Increasing Developable Areas with Turbine Design
The increasing size of wind turbines is a core contributor to lower LCOE, according to the experts in the survey. The cost of land-based wind is expected to fall by, on average, 24% by 2030 and 35% by 2050 under the median (best guess) scenario. Fixed-bottom offshore cost declines are even greater, at 30% by 2030 and 41% by 2015. And costs could be lower for both applications: experts predict a 10% chance that costs will decline by more than 40% by 2030 and more than 50% by 2050.
Though many factors can drive energy cost reductions, turbine size is key. Experts assessed 28 different possible means of reducing LCOE, with the top 5 listed in Table 1. Land-based wind benefits most from increased rotor size (and reduced specific power), rotor design advancements, and increased tower heights. For fixed-bottom offshore wind, the single largest driver is increased turbine generator capacity ratings and rotor diameters, with specific power maintaining current levels.
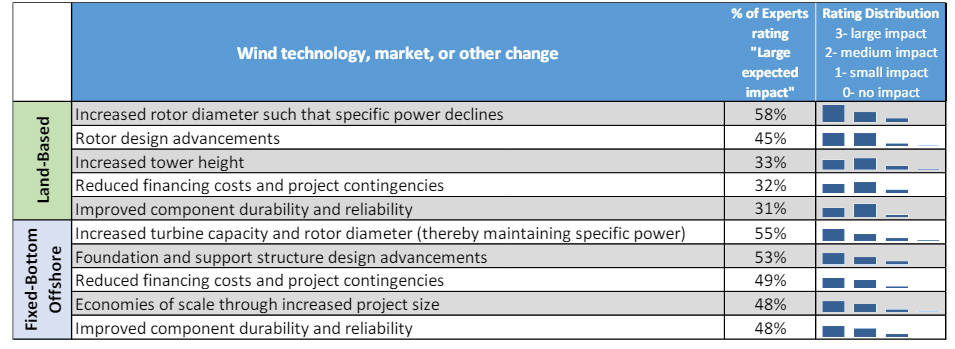
Table 1
The survey also asked how reductions in up-front capital costs (CapEx) and operating expenses (OpEx), increases in capacity factors and turbine design life, and improvements in the cost of financing would affect energy costs.
For land-based wind, experts anticipate that LCOE reductions will be primarily influenced by improvements in capacity factors and CapEx. The taller towers and larger rotors expected to be deployed by 2030 are consistent with the relative importance placed on capacity factor improvements. Achieving lower capital costs while simultaneously increasing turbine size, meanwhile, will require continued design, engineering, and manufacturing advancements.
For fixed-bottom offshore wind, reduced upfront costs are seen as the single most-important driver for LCOE improvements to 2030, with capacity factor increases of somewhat lesser significance. Larger average turbine generator capacities will reduce the high (per-MW) cost of substructures, foundations, and installation experienced offshore. As a report by NREL puts it, “Fewer larger sized turbines minimize the balance-of-system requirements (i.e., less substructures and other infrastructure required to achieve the same project size).” A recent report by BVG Associates, meanwhile, highlights the positive impact of turbine generator size, hub height, and rotor length on not only CapEx, but also OpEx and capacity factor; that report cites a possible 18% reduction in LCOE between 2014 and 2030 based on turbine size alone.
Lower energy costs are not the only benefit from bigger turbines. Recent research shows that machines with low specific power (and taller towers) can boost the value of wind energy in electricity markets, since they have steadier output. Work by and for the U.S. Department of Energy, meanwhile, shows how larger turbines and turbine design advancements might unlock new areas for wind development, both on land and offshore, that would otherwise be deemed unattractive.
Post a Comment:
You may also like:
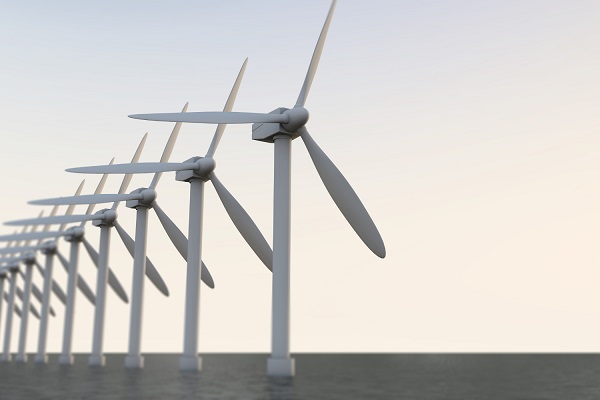
Featured Articles
Economic Impacts of Wind Industry
Any business development will have economic impacts on the local and regional economies, and wind energy projects are no ...
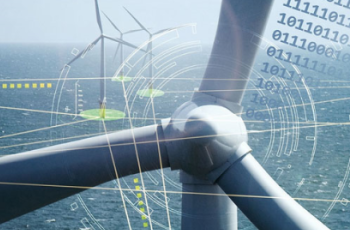
Challenges in Wind Industry
While wind power generation offers numerous benefits and advantages over conventional power generation, there are also some ...
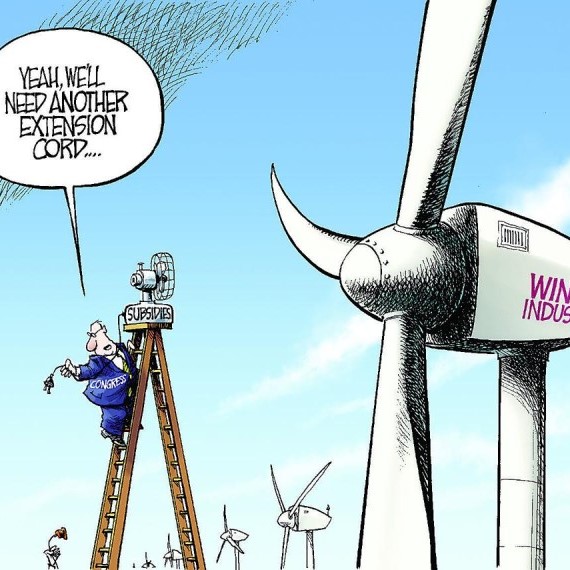
Employment Impacts of Wind Industry
As with most construction and commercial development, wind energy industry create jobs. According to the 2016 Clean Jobs New ...
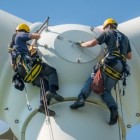
Wind Energy Cost Trends
Though the cost of wind energy has declined significantly since the 1980s, policy measures are currently required to ensure ...
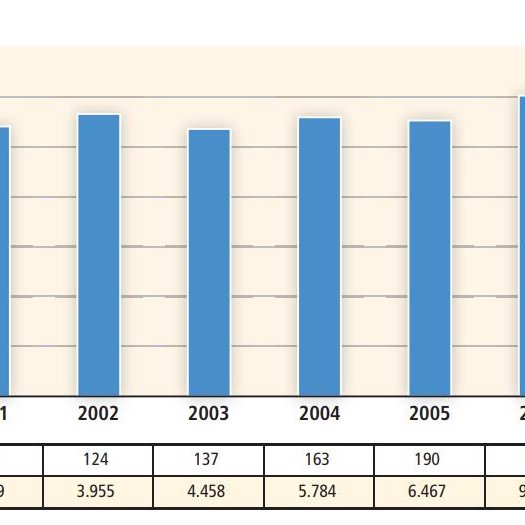
Environmental Impacts of Wind Industry
In recent years, the growth of capacity to generate electricity from wind energy has been extremely rapid, To the ...
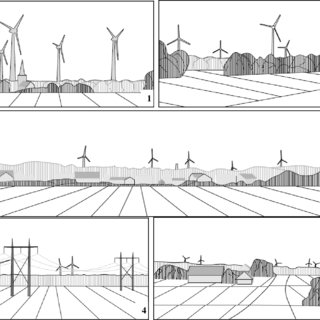